Production of 163Ho
163Ho for ECHo is accessible in thermal neutron activation of 162Er and the subsequent electron capture (EC) decay of the resulting 163Er (T1/2 = 75 min), or in charged-particle induced reactions like p + natDy.
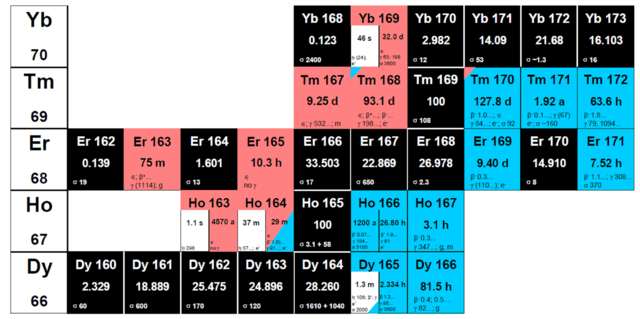
As commercially available samples of any lanthanide element generally contain significant impurities of other lanthanides, unwanted nuclides are inevitably coproduced, with the level depending on the amount of impurities present in the target and the chosen production pathway. Noteworthy is 166mHo, which cannot be separated from 163Ho by chemical means and thus poses a special challenge. Most of the potential impurities have significant -branches, facilitating their detection with
-spectrometry. Thus, after preparation of final samples, characterization with respect to radioactive contamination can be performed via low-level g spectrometry, if desirable also in a low-background environment in an underground laboratory, where detection limits of few tens of mBq/kg are reached.
163Ho production by neutron irradiation of 162Er
Reactor-based production relies on irradiation of Er that is enriched in 162Er. The cross section for the reaction 162Er(n,) → 163Er is 19 barn. Erbium oxide with an isotopic enrichment of 162Er up to about 40 % is commercially available, translates to some 1017 to 1018 atoms of the long-lived 163Ho (T1/2 = 4570 a) that can easily be produced by steady-state irradiation of several tens of mg large Er targets for several weeks in high flux research reactors such as the reactor at the Institute Laue-Langevin in Grenoble, France, at a thermal neutron flux exceeding 1014 cm-2·s-1.
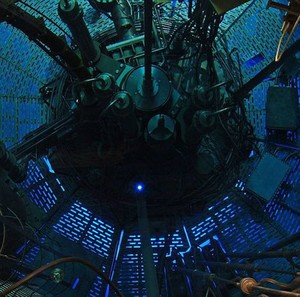
163Ho production by charged particle induced reactions
Alternatively, Dy targets can be irradiated with protons or a-particles to either yield 163Ho directly or as a decay product of 163Er. Due to lower cross sections for this reaction type compared to neutron capture, smaller yields are expected; however, this pathway leads to samples containing smaller amounts of 166mHo than the neutron irradiation pathway.
Currently, the ECHo collaboration expoits the neutron irradiation pathway to produce sufficient amounts of 163Ho.
Purification of 163Ho samples
Within the ECHo project, ultrapure 163Ho samples need to be produced for two different applications: calorimetric measurements of the spectrum following 163Ho electron-capture (EC) decay (ECHo-KIP team) and Penning-trap mass spectrometry for measurements of the QEC-value populating 163Dy (ECHo-MPIK team). The requirements for the corresponding samples differ: calorimetric measurements require radiochemically ultrapure samples, as any nuclear decay unrelated to that of 163Ho will lead to background in the acquired spectra, hence limiting the sensitivity of the measurement. In contrast, in Penning trap mass spectrometry, ions of mass numbers other than 163 will generally not reach the measurement trap (unless they are abundantly present in molecular form with mass 163). However, the QEC-value measurement needs 163Ho samples where the contamination with 163Dy (stable, 24.9 % natural abundance) is very small. To avoid overloading of the purification traps with ions, the ratio of 163Ho/total Ho should not be less than 1/10.
Chemical separation at the Institute for Nuclear Chemistry
To achieve the necessary radiopurity and absence of 163Dy, respectively, chemical separation schemes based on ion-chromatography optimized to separate neighboring lanthanides are used by the ECHo-Mainz team. Separation of Ho from all other lanthanides with high separation factors has already been established, allowing for production of samples, where only lines from 166mHo were visible in the -spectra
Mass separation at the RISIKO mass separator at the Institute for Physics
The presence of 166mHo (T1/2 = 1200 a) in the sample poses a special problem, as chemical approaches cannot provide any elimination of this byproduct, which is especially problematic in calorimetric measurements. Thus, electromagnetic separation is necessary for producing radiochemically ultrapure 163Ho samples without significant traces of 166mHo. The ratio of 163Ho:166mHo depends on the chosen production pathway, but ultimately, a final isotope separation step appears necessary in all those cases, where sufficient 163Ho can be produced in reasonable time. For this, the RISIKO mass separator at the Institute for Physics at the Johannes Gutenberg University Mainz is an ideal tool for further purification of the initial 163Ho sample by efficient and selective suppression of the 166mHo contamination.
RISIKO is a 30-60 kV high transmission mass separator has been set up specifically for elemental ultra-trace determination using resonance ionization mass spectrometric (RIMS) techniques with lasers. As one of the leading development facilities for on-line laser ion sources worldwide, it is equipped with a number of powerful solid-state laser systems for resonance ionization. This combination of laser spectroscopic and mass spectrometric selection ensures both, highest overall ionization and retrieval efficiency for the analyte, in combination with optimum suppression of the contaminating isotope or isobar by many orders of magnitude.
Besides RISIKO, the CERN ISOLDE general purpose separator (GPS) is well suited for mass separation of 163Ho from 166mHo for ECHo.
Sample characterization
Different methods can be employed to characterize the obtained 163Ho samples:
- Neutron activation analysis at the research reactor TRIGA Mainz
- Inductively coupled plasma mass spectrometry
- Isotope ratio analysis at RISIKO
- Autoradiography
-spectrometry
High sensitivity -spectrometric measurements of the radiochemical purity are performed by the low-level facilities in Tübingen and in the Felsenkeller low-level underground laboratory at the TU Dresden, which is located beneath an overburden of 125 m water equivalent of rock.